Non‑ invasive Determination of Hemispheric Language and Upper Limb Dominance in Healthy Subjects
Neinvazívne stanovenie hemisferálnej dominancie rečových funkcií a horných končatin u zdravých subjektou
Úvod:
Problematika vzťahu hemisferálnej dominancie horných končatín a rečových funkcií v zdravej populácii je stále predmetom skúmania a diskusie. Použili sme fokálnu transkraniálnu magnetickú stimuláciu (TMS) ako neinvazívnu vyšetrovaciu metódu k stanoveniu hemisferálnej dominancie končatín a reči a výsledky sme porovnali s viacerými neuropsychologickými testami.
Metodika:
91 zdravých osôb (vek 26 ± 4,6) podstúpilo neuropsychologické testovanie končatinovej dominancie, mapovanie kortikálnej reprezentácie m. abductor pollicis brevis (APB) fokálnou TMS a repetitívnu TMS (rTMS) k stanoveniu lateralizácie rečových funkcií – rTMS(1) s paradigmou číselného radu a rTMS(2) s paradigmou generovania slov.
Výsledky:
Zistili sme štatisticky významnú koreláciu medzi kortikálnou asymetriou reprezentácie ľavého a pravého APB a všetkými neuropsychologickými testami končatinovej dominancie (p < 0,001). Lateralizácia zástavy reči zistená rTMS(1) korelovala s rozhodujúcou väčšinou testov končatinovej dominancie. Pri rTMS(2) sme zistili významne dlhší reakčný čas v 29 zo 42 testovaných subjektoch, ale zistili sme pozitívnu koreláciu s jedným z testov.
Závery:
Končatinová dominancia je združená s asymetriou kortikálnej reprezentácie APB. Pravostranná dominancia reči v skupine zdravých pravákov nie je taká zriedkavá, ako sa myslí (9,5 %). Čím je výraznejšia ľavorukosť, tým vyššia je pravdepodobnosť lateralizácie rečových funkcií v pravej hemisfére (9,5– 33 %). Použitím rTMS sme ďalej zistili: a) tvorba reči môže byť ovplyvnená v dvoch rôznych miestach gyrus frontalis inferior, b) pars triangularis je zavzatá do verbálnej produkcie iba u niektorých jedincov, c) motorický prah je významne vyšší pre kognitívne ako pre motorické funkcie. TMS s použitím fokálnej cievky môže byť objektívnou metódou k určeniu končatinovej a rečovej hemisferálnej dominancie.
Kľúčové slová:
končatinová dominancia – rečová dominancia – hemisferálna lateralizácia – transkraniálna magnetická stimulácia
Authors:
M. Turcanova Koprusakova 1; E. Kurca 1; S. Sivak 1; M. Bittsansky 2; M. Benco 3; D. Sutorova 1; K. Javorka 4; H. Polacek 5
Authors‘ workplace:
Clinic of Neurology, Jessenius Faculty of Medicine, Comenius University and University Hospital, Martin
1; Institute of Medical Biochemistry, Jessenius Faculty of Medicine, Comenius University, Martin
2; Department of Neurosurgery, University Hospital, Martin
3; Institute of Physiology, Jessenius Faculty of Medicine, Comenius University, Martin
4; Clinic of Radiology, Jessenius Faculty of Medicine, Comenius University, Martin
5
Published in:
Cesk Slov Neurol N 2013; 76/109(3): 315-321
Category:
Original Paper
Autoři deklarují, že v souvislosti s předmětem studie nemají žádné komerční zájmy.
Redakční rada potvrzuje, že rukopis práce splnil ICMJE kritéria pro publikace zasílané do biomedicínských časopisů.
Overview
Introduction:
There still is some debate about the relationship between handedness and speech in healthy subjects. We used focal transcranial magnetic stimulation (TMS) as a non‑invasive method to determine hemispheric dominance and compared our results with several neuropsychological tests.
Methods:
91 healthy subjects (age 26 ± 4.6) underwent neuropsychological testing for handedness, TMS mapping of cortical representation of abductor pollicis brevis (APB), repetitive TMS (rTMS) to determine language lateralization with Number‑ counting paradigm – rTMS(1), and Verb‑ generation paradigm – rTMS(2).
Results:
There was a significant correlation between cortical asymmetry of the left/ right APB and all the used neuropsychological tests of handedness (p < 0.001). Laterality of speech arrest using rTMS(1) correlated with the majority of tests for handedness. When rTMS(2) was used, there was significantly longer reaction time in 29/ 42 subjects but we found correlation with only one of the tests.
Conclusion:
Handedness is associated with asymmetry in cortical motor representation. Right‑ cerebral dominance for language in healthy right‑ handers is not as rare as it was supposed (9.5%). Stronger left‑ handedness is associated with higher probability of language dominance in the right hemisphere (9.5–33%). When using rTMS, we found that: a) speech production can be influenced by 2 different areas in the gyrus frontalis inferior, b) pars triangularis is involved in verb production only in some subjects, c) motor threshold is significantly higher for cognitive processes than for motor functions. TMS with focal magnetic coil can be used as an objective method for mapping cortical motor asymmetry of handedness and language functions.
Key words:
handedness – language – functional laterality – transcranial magnetic stimulation
Abbreviations
APB | Abductor Pollicis Brevis |
EEG | ElectroEncephaloGraphy |
EHIT | Edinburgh Handedness Inventory Test |
IFG | Inferior Frontal Gyrus |
IS | Stimulus Intensity |
MEP | Motor Evoked Potential |
MRI | Magnetic Resonance Imaging |
MT | Motor Treshol |
MZT | Matejcek‑ Zlab Test |
NHPT | Nine‑ Hole Peg Test |
TMS | Transcranial Magnetic Stimulation |
rTMS | repetitive Transcranial Magnetic Stimulation |
RT | Reaction Time |
Introduction
Transcranial magnetic stimulation (TMS) has become a powerful technique for investigating brain functions. Application of TMS to cognitive functions provides a unique opportunity to create a transient functional lesion in a normal brain to determine whether a brain area is required for completion of a given task. Magnetically induced speech arrest is one of the most dramatic examples of TMS. Several groups have now reported that rTMS over the left frontal or either the left or right motor cortex can cause speech arrest, stutter or word segments repetition [1– 4]. Studies in which neurolinguistic questions are addressed with TMS, rather than just the possibility of disrupting speech output, are now being conducted [5– 8]. Since Broca described the association between language production and brain lesions of the left hemisphere in 1861, it has been widely accepted that language production in right‑ handed subjects is usually a function of the left hemisphere [9]. However, lateralization of language is not as well defined in left‑ handed people as it is in their right‑ handed counterparts [10]. Luria was among the first who pointed out that this association might not be universally true since even in left‑ handers, aphasia frequently occurs as a result of a left hemisphere lesion [11]. A growing body of evidence suggests that a functional link exists between the hand‑ motor area of the language‑ dominant hemisphere and the regions responsible for language processing. All authors who explored language or motor functions had usually investigated a small group of either healthy or unhealthy subjects.
The aim of our study was to determine hemispheric dominance for handedness and language with TMS on a large group of young, healthy subjects. We used a single focal TMS with a figure‑of‑ eight‑ shaped magnetic coil to find asymmetry in cortical motor representation of abductor pollicis brevis (APB) muscles in right‑ handed and left‑ handed subjects, and to determine language lateralization with repetitive stimulation (rTMS). Our results of TMS were compared with several different neuropsychological tests for handedness.
Material and methods
Subjects
The study involved 91 healthy volunteers (30 men, mean age 25.97 ± 4.4 years; 61 women, mean age 26.7 ± 4.6 years). Based on the writing hand there were 74 right‑ handed (22 men, 52 women) and 17 left‑ handed subjects (8 men, 9 women). None of the subjects had a history of any neurological disorders, including seizures or brain injury. The local ethics committee approved the protocol and all subjects gave their informed consent.
Neuropsychological tests
We quantified hand preference in each subject using several tests:
- The Edinburgh Handedness Inventory Test [12] – subjects reported their hand preference – right, left, ambidexterity in response to 10 questions (e. g. Which hand do you use to throw a ball? Write? Eat with spoon?...)
- Test of laterality by Matejcek‑ Zlab [13] – the examiner asked a subject to do certain activities and noted the hand he/ she preferred – e. g. put a key in a keyhole, put beads into a box, throw a ball into a box, thread a needle, touch their ear etc.). Hand preference was expressed by coefficient of right‑ handedness (DxQ) – number of right‑ handed reactions in percentage – D × Q = (R + A/ 2)/ N × 100 – this means that a sum of right‑ handed reactions (R) plus half of ambidextrous reactions were divided by the number of all activities (N) multiplied by 100. Results of the test could be as follows: DxQ 100– 90 – strong right‑ handedness, DxQ 89– 75 – moderate right‑ handedness, DxQ 74– 50 – mixed handedness or ambidexterity, DxQ 49– 25 – moderate left‑ handedness, DxQ 24– 0 – strong left‑ handedness.
- The Nine‑ Hole Peg Test is a simple, timed test of fine motor coordination. Reliability and validity have been assessed and normal values are available [14]. The test involves a subject placing 9 pegs in 9 holes. Subjects are scored on the length of time it takes to place and remove all 9 pegs [15,16]. The results from all neuropsychological tests were compared and correlations between them were explored.
TMS mapping of acral hand muscles
Subjects were seated comfortably in an armchair in a quiet room. Motor‑ evoked potentials (MEPs) were monitored with EMG surface electrodes placed over left and right APB. Muscle response was recorded on relaxed muscles. We used the Nicolet Viking II E electromyograph and a Magstim Rapid magnetic stimulator (Magstim Co, UK) with a figure‑of‑ eight‑ shaped magnetic coil (type 9925, diameter 70 mm). We were mapping cortical muscle representation using modified Triggs‘ method [17]. We first stimulated the right and then the left hemisphere, the coil was moved 1 cm apart. We delivered three stimuli to each of the stimulated sites and, using Viking II nerve conduction software, we determined the mean amplitude of rectified MEPs. A cortical area of the right and left APB muscle was determined by the number of scalp stimulation sites eliciting MEPs with more than 10% of the maximum amplitude.
rTMS and hemispheric language dominance
To determine the hemispheric language lateralization, we used rTMS and two different paradigms – 1. Number‑ counting paradigm – rTMS(1) and 2. Verb‑ generation paradigm – rTMS(2). The stimulation parameters were chosen according to the current safety guidelines for rTMS [18,19].
Number‑counting paradigm – rTMS(1)
The speech test was performed in a seated position using anterior‑ posterior coil orientation, with stimulus intensity set initially to hand motor threshold (MT) and the repetition rate to 4 Hz [20]. We started with supramaximal intensity of stimuli (110% of MT) for the APB muscle [21]. If no speech effect was obtained, intensity was increased by 5% of motor threshold up to a maximum of 150% for 10 s. A complete speech arrest was defined as an absence of any structural or recognizable language but not necessarily sound cessation [3]. Stimulation was stopped as soon as total speech arrest was observed. Evaluation of the speech area was performed without knowing the handedness.
Verb‑generation paradigm – rTMS(2)
We applied an acoustic verb‑ generation paradigm with 4 replications [9]. The test consisted of a rest period and a verb‑ generation task (finding a semantically matching verb for each noun read aloud). We used pars triangularis of the inferior frontal gyrus (IFG) as the target. The stimulation sites were identified on 3- dimensional MRI T1- weighted images (1.5T Magneton Symphony Maestro Class, Siemens AG, Germany) of the brain and the optimum site of stimulation was marked on the scalp with a software system Magic View 300. We measured the distance between the lateral end of the eyelid (A) and the tragus (B), and marked the centre of pars triangularis as a perpendicular line to AB distance. rTMS was performed with Magstim rapid stimulator (2.1 T) with a 70- mm figure‑of‑ eight coil and supramaximal intensity 110% of MT for APB at the frequency of 4Hz. We stimulated left and right side of the pars triangularis and the vertex (Cz according 10– 20 system EEG) as a control. A list of nouns was used for the generation task, with a two‑minute rest between the stimulations. The nouns were selected from a list of high‑frequency Slovak nouns. The voice of the investigator and the patient were recorded digitally, and the reaction time latencies were measured with a freely available software package (Quartz AudioMaster). The authors declare that experiments on human subjects reported in the manuscript was performed in accordance with the ethical standards of the responsible committee on human experimentation (institutional and national) and with the Helsinki Declaration of 1975, as revised in 2000.
Results
Neuropsychological tests
The ratio of left‑ / right‑ handers and ambidexters was slightly different depending on the test used. However, there were significant correlations (NHPT and EHIT – r = 0.58, p = 1.9 × 10–9, NHPT and MZTb – r = 0.56, p = 7.1 × 10–9, EHIT and MZTb – r = 0.89, p = 9.6 × 10–33, NHPT and MZTa r = 0.51, p = 2.83 × 10–7, EHIT and MZTa – r = 0.75, p = 1.36 × 10–17). MZTa means that moderately right‑ and left‑ handed (DxQ 89– 75 and DxQ 49– 25) were included in the ambidexter group (DxQ 74– 50), while MZTb means that moderately right‑ handed were included in the right‑ hander group, and moderately left‑ handed were in the left‑ hander group.
TMS mapping of acral hand muscles
In 91 subjects we used a focal magnetic coil with supramaximal intensity of stimuli (110% of MT for APB – 65% ± 9%). We found that the number of scalp stimulation sites eliciting MEPs (MEPs higher than 10% of the maximum eliciting amplitude of MEP) was statistically greater for APB muscles of the preferred hand (preferred – 13.2 ± 5.3 vs non‑preferred – 9.4 ± 4.6, p = 1.43 × 10–7, because of non‑homogenic distribution we used non‑parametric Wilcoxon test and Spearmann correlation coeficient). Our results significantly correlated with all used neuropsychological tests for handedness. (NHPT – p = 2.5 × 10–4, MZT – p = 0.002, EHIT – p = 2.96 × 10–4, Pearson correlation coefficient).
rTMS and language functions – number‑counting paradigm
A group of 45 healthy subjects underwent rTMS while counting numbers. Speech arrest occurred in 42/ 45 subjects (6 – stimulation of right hemisphere, 29 – left hemisphere, 7 – bilaterally). In 3 subjects, there was no effect of rTMS on speech with the maximum intensity of 150% of MT. Speech arrest site was defined in relation to Cz using two axes (axis x – a connecting line of tragus and Cz; axis y – a connecting line of nasion, Cz and inion perpendicular to axis x): in the left hemisphere – x = 9.17 ± 0.6 cm, y = 4 ± 0.54 cm, and in the right hemisphere – x = 9.25 ± 0.5 cm, y = 4 ± 0.0 cm. The intensity of TMS causing speech arrest was significantly higher than the one causing motor response of APB, n = 42, IS for speech arrest – 74% ± 12%, for motor response of APB – 63% ± 9%, p = 4.59 × 10–6). Speech arrest was induced in the left hemisphere in 71.4% subjects, in the right in 11.9% and in both hemispheres in 16.7% subjects.
rTMS and Verb‑generation paradigm
We examined 42 healthy subjects. The mean intensity of stimuli was 57% ± 5%. The optimum position of stimulation (pars triangularis) was localized at 31.9 ± 3.2 mm from the lateral end of the eyelid and at 45 ± 3.4 mm on the left hemisphere and 44.3 ± 3.6 mm on the right up from the baseline on perpendicular line. We did not observe any qualitative effects such as non‑responses or verb‑ generation dysfunction during rTMS stimulation in any subject. There was a significant increase of reaction time latency in all subjects during the stimulation as compared to no stimulation (p < 0.01). To eliminate stimulation effect of TMS on reaction time we chose the vertex as a control point and compared the latencies in stimulated sites of pars triangularis on the left and right sides. We evaluated latency with the second series of verbs. Latency increase of RT (ms) during TMS was considered significant if at least three latencies were above the 75th percentile of the vertex stimulation.
Reaction time latencies were significantly longer (p < 0.01) over pars triangularis relative to vertex in 29/ 42 subjects; we did not find significant difference in the remaining 13 subjects. In 15 subjects, language function was localized in the left hemisphere (51.7%), in the right in 5 (17.2%) and bilaterally in 9 (31.1%).
Relationship between handedness and language
We determined that there is a relationship between speech and language functions (rTMS1 and rTMS2) and handedness using neuropsychological tests as well as objective TMS mapping of acral hand muscles (Tab. 1– 3). We used Spearman correlation coefficient. We found a strong correlation between lateralization of rTMS(1) speech arrest and handedness: 1. Language to NHPT (ro = – 0.42, p = 0.005), 2. Language to EHIT (ro = – 0.47, p = 0.002), 3. Language to MZTa (ro = – 0.27, p = 0.08), Language to MZTb (ro = – 0.41, p = 0.008), 4. Language to acral hand mapping (ro = 0.34, p = 0.028). rTMS(2) correlated with one test only: 1. Language to NHPT (ro = 0.48, p = 0.01), 2. Language to EHIT (ro = 0.3, p = 0.12), 3. Language to MZTa (ro = 0.39, p = 0.11), Language to MZTb (ro = 0.3, p = 0.11), 4. Language to acral hand muscles (ro = 0.3, p = 0.11). Relationships between language lateralization and handedness are illustrated in Fig. 1–3.
Discussion
We confirmed a significant asymmetry of cortical motor representation for APB muscle between the dominant and non‑dominant upper limb using focal TMS. Our results suggest that there is a statistically significant correlation between handedness and cortical asymmetry in the upper limb muscle representation. It seems less unlikely that technical or methodological factors influenced our results since we examined a relatively large set of healthy subjects (n = 91) and our results significantly correlated with other tests for handedness. Hemispheric dominance for motor functions can be determined by subjective (EHIT) and/ or by objective (MZT, NHPT, acral hand muscles mapping with TMS) methods. Focal TMS represents a unique non‑invasive objective method with well‑defined quantitative parameters to determine handedness.
We determined lateralization of speech and language functions using low‑ frequency rTMS at the rate of 4 Hz. We used two different paradigms – number‑ counting paradigm (rTMS(1)) and verb‑ generation paradigm (rTMS(2)). We induced expressive aphasia in 42 of 45 people using rTMS(1). An average stimulus intensity (IS) needed to affect language functions was significantly higher than the intensity for motor function investigations (IS for speech – 74% ± 12%, IS for motor functions – 63% ± 9%). Aphasia was induced most frequently using IS at 120% MT APB (10 of 42 subjects), 110% MT APB (9 of 42 subjects), and 115% MT APB (6 of 42 subjects). In other cases (17 of 42 subjects), even higher IS (with range 125%– 145%) was used. Using low‑ frequency rTMS we found that approximately 9% of healthy right‑ handed subjects have so‑ called Broca’s area (the area essential for language functions) located paradoxically in the right hemisphere. Lateralization of speech and language functions in the right hemisphere ranges from 9.5% in the group of strong right‑ handers up to 33% in the group of strong left‑ handers. The fact, that we examined a set of only healthy individuals, allows us to confirm the statement, that speech lateralization in the right hemisphere is a natural phenomenon, not a consequence of pathological disease processes.
Our results are comparable with other authors who determined speech lateralization by rTMS at a frequency of 3 Hz [21]. They induced speech arrest most frequently with IS at 140% MT. In our study, we stimulated with the 4 Hz frequency and speech arrest was seen most often at 120% MT. This supports the argument that lower stimulation frequency requires higher IS to induce aphasia [20]. Epstein et al [20] systematically investigated the effects of both intensity and rate of stimulation on speech arrest in healthy individuals. He found that speech arrest could be obtained with rTMS repetition rate as low as 2 Hz, intensity of 150% motor threshold or less and pulse trains of no more than 5 s. As expected, higher intensities led to stronger speech arrest effects but, surprisingly, it was the lower rates of stimulation (4– 8 Hz) that were more reliable at inducing speech arrest than those used in previous studies (16– 32 Hz; [1,2]). Higher frequencies led to prominent facial and laryngeal muscle contractions and significantly increased discomfort or pain associated with stimulation, making speech arrest more difficult to determine.
We declare that low‑ frequency rTMS (4Hz) is safe to use and offers an alternative to high‑frequency stimulation rTMS with only a minor, well‑tolerated discomfort for the subjects (mild to moderate pain during the stimulus due to pericranial muscle contraction). Our results show, that lower intensity stimulations (sufficient to stimulate upper limb muscles) are not sufficient to inhibit speech and language functions. The threshold IS for low‑ frequency rTMS sufficient for inhibition of complex frontal executive speech centre is higher than the threshold for induction of motor upper limb response (p = 4.59 × 10–6).
Verb‑ generation paradigm methodology (rTMS(2)) was used in 42 healthy subjects. For comparison of reaction times (RT) during stimulation of the right and left hemispheres we used the same approach to evaluating treatment results as previous authors [22]. They compared prolongation of RT with and without stimulation. We modified their method so that we used RT from vertex stimulation as a control. This eliminates the effect of stimulation on RT as confirmed by a significantly shorter RT latency without stimulation with this method. We evaluated the second set of verbs. During bilateral stimulation of perisylvic area, RT was significantly prolonged (p < 0.01) when compared to RT during vertex stimulation (29 of 42 subjects). We obtained very similar results using two different rTMS methods for determining speech and language centre location. Localization of speech and language functions in the right hemisphere increased from about 9% in strong right‑ handed subjects to about 30% in strong left‑ handers.
Our results suggest that speech and language functions can be influenced from two different frontal locations. Stimulation of the posterior location – rTMS(1) – causes speech arrest. During rTMS(2), we stimulated pars triangularis area that is localized further forward. We expect that the stimulated dorsal area corresponds with the ventral part of the precentral gyrus, where motor and premotor representations of phonatory muscles are located. In other words, stimulation causes speech arrest due to interference with the motor component of speech. Stimulation of the pars triangularis (part of Broca‘s centre and precentral cortex) causes interference with planning of the articulation, as manifested by prolongation of the reaction time. Both considered mechanisms of speech production in the two stimulated areas are mostly localized monohemispherally in normal population. In line with this, other works have shown that speech arrest can be induced from the front or the rear part of inferior frontal gyrus [5,23]. Other authors presume that specialized language functions are phylogenetically located in perisylvian area, where the whole motor‑ effector system for the creation of oral‑ buccal‑lingual‑ vocal sequences is located [24,25]. Epstein and co‑ authors stated that fast and precise coordination between oral‑ buccal‑lingual‑ vocal movements and the formation of long sequences (so‑ called phonemes) is one of the most extraordinary and the most complex tasks carried out by the human motor system [20]. This explains, among other things, the elective vulnerability of speech production and language functions to the inhibitory low‑ frequency 4Hz rTMS. Our results support the current interpretation of speech and language functions in terms of a so‑ called network module. This is in contrast to the original conception of one frontal speech centre. We expect that various individual functional speech units have certain and significant structural and anatomical overlap. We also assume that, while the chosen muscles and muscle groups are represented bilaterally, the network module for speech generation and for language functions in the anatomical area is mostly located unilaterally. It follows that rTMS in this location results in speech inhibition not only in complex and long phonemes but also in acquired automatic speech production in form of numerical series. Stimulation of pars triangularis in Verb‑ generation paradigm at IS 110% MT led to significant RT prolongation in 29 out of 42 examined individuals. On the other hand, significant prolongation of RT was not present in 13 of 42 people. This can be because a specific anatomical structure (e. g. pars triangularis) might not necessarily be involved in a particular function (e. g. verb production) in each individual. This is consistent with studies that used Verb‑ generation paradigm and a functional imaging method. In some of their subjects, the activated area did not correspond to the expected anatomical structure [9,22]. Moreover, the inhibitory rTMS of Broca‘s area did not suppress verb production specifically but influenced general speech production [26]. Another explanation could be that, in some patients, IS at 110% MT was not sufficient to induce the inhibitory effect.
Functional imaging methods currently dominate studies of cerebral localization for various functions. However, problems might occur with interpretation of results. The key issue is to assess the true causal relationship between activated brain regions and a specific task. rTMS offers a useful complementary method to neuropsychological testing and imaging methods to study cerebral function localization in a non‑invasive way.
Conclusion
Handedness is associated with asymmetry in cortical motor representation of acral upper limb muscles. Focal transcranial magnetic stimulation can be used as an objective method for mapping of cortical motor asymmetry of upper limbs and for assessment of handedness. Cortical mapping, using inhibitory TMS, enables us to determine whether a certain brain area is involved in speech production and is required for performing a certain language function. Our results support the theory that a complex frontal executive speech centre is not an anatomically well‑defined area but it is a network module consisting of several functional units. The network module is usually unilateral and its localization can vary between individuals. The study also shows that there is a relationship between the cortical motor area for the more‑or‑ less dominant upper limb and the cortical areas for speech and language functions. Stronger left‑ handedness is associated with higher probability that the speech and language areas are located in the right hemisphere. Since we examined healthy individuals only, we can conclude that speech and language function lateralization in the right hemisphere is a natural phenomenon and not a consequence of pathological processes. We have also validated the finding, that lateralization of language functions in the right hemisphere is not as rare as previously assumed. TMS and rTMS are simple non‑invasive methods that enable examination of particular brain functions.
The authors declare they have no potential conflicts of interest concerning drugs, products, or services used in the study.
The Editorial Board declares that the manuscript met the ICMJE “uniform requirements” for biomedical papers.
Prof. Egon Kurca, MD, PhD.
Neurology Clinic
Jessenius Faculty of Medicine
University Hospital
Kollarova 2
036 01 Martin
e-mail: egonkurca@gmail.com
Accepted for review: 20. 8. 2012
Accepted for print: 21. 12. 2012
Sources
1. Pascual‑ Leone A, Dhuna AK, Gates JR. Study of the frontal speech area with rapid‑ rate transcranial magnetic stimulation. Electroencephalogr Clin Neurophysiol 1991; 85: 25.
2. Jennum P, Friberg L, Fuglsang‑ Frederiksen A, Dam M.Speech localization using repetitive transcranial magnetic stimulation. Neurology 1994; 44(2): 269– 273.
3. Epstein CM, Lah JK, Meador K, Weissman JD, Gaitan LE, Dihenia B. Optimum stimulus parameters for lateralized suppression of speech with magnetic brain stimulation. Neurology 1996; 47(6): 1590– 1593.
4. Flitman SS, Grafman J, Wassermann EM, Cooper V,OʼGrady J, Pascual‑ Leone A et al. Linguistic processing during repetitive transcranial magnetic stimulation. Neurology 1998; 50(1): 175– 181.
5. Stewart L, Walsh V, Frith U, Rothwell JC. TMS produces two dissociable types of speech disruption. Neuroimage 2001; 13(3): 472– 478.
6. Shapiro KA, Pascual‑ Leone A, Mottaghy FM, Gangitano M, Caramazza A. Grammatical distinctions in the left frontal cortex. J Cogn Neurosci 2001; 13(6): 713– 720.
7. Shapiro K, Caramazza A. Grammatical processing of nouns and verbs in left frontal cortex? Neuropsychologia 2003; 41(9): 1183– 1198.
8. Meister IG, Boroojerdi B, Foltys H, Sparing R, Huber W, Töpper R. Motor cortex hand area and speech: implications for the development of language. Neuropsychologia 2003; 41(4): 401– 406.
9. Thiel A, Habedank B, Herholz K, Kessler J, Winhuisen L, Haupt WF et al. From left to the right: how the brain compensates progressive loss of language function. Brain Lang 2006; 98(1): 57– 65.
10. Geschwind N, Galaburda AM. Cerebral lateralization. Biological mechanism, associations and pathology: I. A hypothesis and a program for research. Arch Neurol 1985; 42(5): 428– 459.
11. Luria AR. Cognitive development: Its cultural and social foundations. Cambridge: Harvard University Press 1976.
12. Oldfield RC. The assessment and analysis of handedness: the Edinburgh inventory. Neuropsychologia 1971; 9(1): 97– 113.
13. Matejček Z, Žľab Z. Zkouška laterality. Psychodiagnostické a didaktické testy. Bratislava: Psychodiagnostika 1972: 1– 19.
14. Oxford Grice K, Vogel KA, Le V, Mitchell A, Muniz S,Vollmer MA. Adult norms for a commercially available Nine Hole Peg Test for finger dexterity. Am J Occup Ther 2003; 57(5): 570– 573.
15. Minks E, Mareček R, Pavlík T, Chroust K, Bareš M.Vliv repetitivní transkraniální magnetické stimulace cerebella na motoriku horní končetiny u pacientú v iniciálním stadiu Parkinsonovy nemoci – pilotní studie. Cesk Slov Neurol N 2010; 73/ 106(1): 32– 36.
16. Minks E, Marecek R, Pavlik T, Ovesna P, Bares M. Is the cerebellum a potential target for stimulation in Parkinsonʼs disease? Results of 1- Hz rTMS on upper limb motor tasks. Cerebellum 2011; 10(4): 804– 811.
17. Triggs WJ, Subramanium B, Rossi F. Hand preference and transcranial magnetic stimulation asymmetry of cortical motor representation. Brain Res 1999; 835(2): 324– 329.
18. Wassermann EM. Risk and safety of repetitive transcranial magnetic stimulation: reported and suggested guidelines from the International Workshop on the Safety of Repetitive Transcranial Magnetic Stimulation, June 5– 7, 1996. Electroencephalogr Clin Neurophysiol 1998; 108(1): 1– 16.
19. Tassinari CA, Cincotta M, Zaccara G, Michelucci R. Transcranial magnetic stimulation and epilepsy. Clin Neurophysiol 2003; 114(5): 777– 798.
20. Epstein CM, Meador KJ, Loring DW, Wright RJ, Weissman JD, Sheppard S et al. Localization and characterization of speech arrest during transcranial magnetic stimulation. Clin Neurophysiol 1999; 110(6): 1073– 1079.
21. Khedr EM, Hamed E, Said A, Basahi J. Handedness and language cerebral lateralization. Eur J Appl Physiol 2002; 87(4– 5): 469– 473.
22. Winhuisen L, Thiel A, Schumacher B, Kessler J, Rudolf J, Haupt WF et al. The right inferior frontal gyrus and poststroke aphasia: a follow‑up investigation. Stroke 2007; 38(4): 1286– 1292.
23. Aziz‑ Zadeh L, Cattaneo L, Rochat M, Rizzolatti G. Covert speech arrest induced by rTMS over both motor and nonmotor left hemisphere frontal sites. J Cogn Neurosci 2005; 17(6): 928– 938.
24. Ojemann GA, Mateer C. Human language cortex: localization of memory, syntax, and sequential motor‑ phoneme identification systems. Science 1979; 205(4413): 1401– 1403.
25. Ojemann GA. Cortical organization of language. J Neurosci 1991; 11(8): 2281– 2287.
26. Cappelletti M, Fregni F, Shapiro K, Pascual‑ Leone A, Caramazza A. Processing nouns and verbs in the left frontal cortex: a transcranial magnetic stimulation study. J Cogn Neurosci 2008; 20(4): 707– 720.
Labels
Paediatric neurology Neurosurgery NeurologyArticle was published in
Czech and Slovak Neurology and Neurosurgery
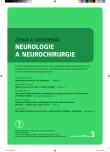
2013 Issue 3
Most read in this issue
- Mechanisms of Spasticity and its Assessment
- Human Prion Diseases in the Czech Republic – 10 Years of Experience with the Diagnosis
- Inclusion Body Myositis with Neck Muscle Weakness and Positive Effect of Immunoglobulins – a Case Report
- Extracranially Metastasizing Meningiomas